There are many great testaments to humanity's perseverance. The Pyramids at Giza

, the Cathedral of Notre Dame

, the Great Wall of China

, and the most recent
Applied Biophysics for Drug Discovery 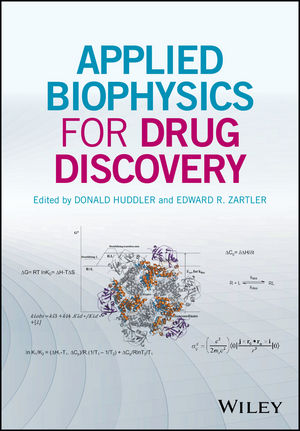
. This blog typically
reviews the content of the book, but I thought it would be interesting to describe how the book came together.
It is said that if you see
inside a hotdog factory
you'd never eat a hotdog again. So, how did this hotdog get made? Flash back 3 years, and Don Huddler (at that time at
GSK) reaches out to me and says, "Hey do you have a contact at Wiley. I
have a great idea for a book." "Sure Don, here you go."
A week or so later, Don invites me to lunch so we go to
greatest restaurant ever!!! Over lunch , and maybe my second beer, Don says, lets write this together. After almost choking on my Cajun Meatloaf GC, I said NO WAY!!! There may have been at least one more beer involved but I agreed after Don said he would do all the heavy lifting.
Flash forward a few weeks, and Wiley accepted our book proposal, with 30 listed chapters, with 5 sections. Part of the fun part is that they send your proposal out for review, so just like a paper, you get reviewer comments. You then sign a contract with the Publisher that says you will deliver so many pages: 556 in our case by
31Jan2016 (14 months after the contract was signed
). Don had already defined many of the authors who he had thought would be appropriate. So, at this point, you send out letters to authors and ask them to contribute. So, "You would be perfect to write a chapter for our upcoming book on
[insert what you want them to write on]." Then you wait and hope that they actually respond. Many authors responded right away, and it is not personal when they say no. It is a pretty significant amount of work to contribute to a chapter. Then you work with the authors to define the time frame of when drafts are due, and so forth.
You then wait and hope the authors deliver on time. Some do, may don't. So then you become Nagger-in-Chief. "Where's the chapter draft? Is it coming soon?" As someone famous said, life is what happens while you are making other plans. During the summer of 2015, GSK was re-orging, and Don decided to go to Law School full time. So, here we are trying to get drafts done, edited and returned to authors and one editor makes a major career change. Two months after that, I followed Don in changing careers, leaving the glamorous consulting life to join Pfizer. As hard as we tried, our new careers required our focus and the book got short shrift.
For some authors who had delivered on time, this was frustrating. We had a given deadline and they delivered. We dropped the ball for them. For other authors who were less than timely in their contributions, they did not get nagged sufficiently, causing further delays. 2016 was by and large a horrible year if you were an author with a delivered chapter, and a fantastic year if you hadn't delivered a chapter yet. And of course, as time drags on, other career changes happen. One primary author retired, one changed jobs and stopped responding to emails, and so on. So, our initial 20 chapters (from the 30 we wanted) was whittled down to 15 chapters.
Its now 2017, and the book is a year late, and Don and I are still trying the best we can to manage new careers and editing the book. Authors are angry with the delay and I didn't blame them. We were actually able to finally get all the chapters together and to the publisher. Phew, most of our work is done. STOP! One chapter got completely left out of the final submission. So, the scramble was on to make sure that it was included. You can see which one when you try to figure out the order of chapters.
So, what is the role of the Publisher during this whole thing you ask? I don't know. My experience with the publisher on this book was VERY different from the first one. I got the feeling that this book was a low priority for them. Our emails took weeks sometimes to be responded to. It was frustrating; but something we did not want to share this with the authors. So, after this it is mostly on the publisher, but we had to design the cover. To explain it, everything on the cover is from a chapter in the book: the structure and equations. We get to galley proof stage and final publication, and Wiley tells us we need to index the book. This is a change from the first time. Don and I take the option to have them do it for us and take it out of royalties (which is something on the order of 50$ a year). So, finally in July of this year, it was finalized and given a publication date. Project done. So, let me say to all the authors, thank you so much for being a part of this book.
So, what did I learn co-editing this book? No matter how many beers I get fed, I will never EVER edit a book again. There is actually a word
ambit. I have a reputation for being "provocative" (from a reviewer);
this probably didn't help. The quality of the contact at the publisher makes a huge difference. I hope our contact at Wiley was new and inexperienced because this experience was far worse than the first time I did the book.
So, what is inside the book? 291 pages (out of a promised 556). There are 14 chapters of awesomeness, featuring people featured on this site previously. This book is focused on biophysical methods and how they are used to triage and advance leads. Many of these topics have been covered in depth on this site:
thermodynamics,
protein-protein interactions,
HDX,
MST,
SPR,
WAC,
1D NMR, Protein
NMR, and how to use them in terms of
residence time. There are two case studies, one from Pfizer and one from FOB (Friend of the Blog) Michelle
Arkin. Lastly, and you can figure this out, there is a chapter from Martin
Scanlon on fragment
libraries. I won't go into actually reviewing the book; that would be a major conflict of interest (I do have a financial interest in it and Don and I need to pay for that indexing!).
I would appreciate comments on it here, and of course any other questions I would be happy to answer.